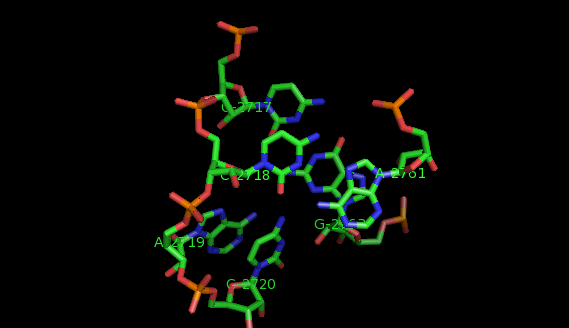
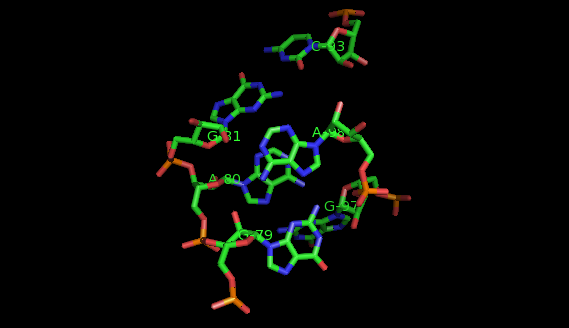
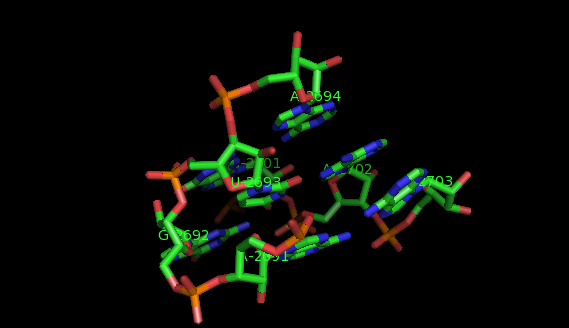
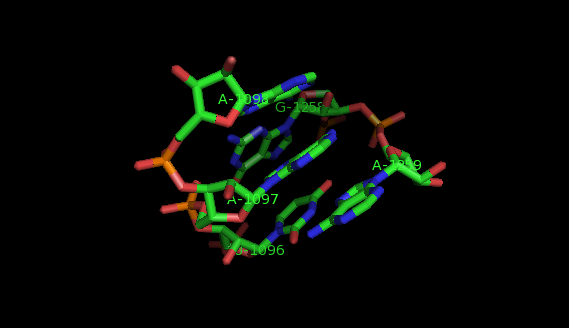
What are motifs?
RNA (Acronym for RiboNucleic Acid) is a family of nucleic acids very similar to DNA, but differs in a few important structural details: in the cell RNA is usually single stranded, while DNA is usually double stranded. RNA nucleotides contain ribose while DNA contains deoxyribose (a type of ribose that lacks one oxygen atom), and in RNA the nucleotide Uracil substitutes for Thymine which is present in DNA.
Several types of RNA are known. Messenger RNA (mRNA) is the RNA that carries information from DNA to the ribosome, the sites of protein synthesis (translation) in the cell. The coding sequence of the mRNA determines the amino acid sequence in the protein that is produced. Many RNAs do not code for protein however. These non-coding RNAs can be encoded by their own genes (RNA genes), but can also derive from mRNA introns. The most prominent examples of non-coding RNAs are transfer RNA (tRNA) and ribosomal RNA (rRNA), both of which are involved in the process of translation. There are also non-coding RNAs involved in gene regulation, RNA processing and other roles. Certain RNAs are able to catalyse chemical reactions such as cutting and ligating other RNA molecules, and the catalysis of peptide bond formation in the ribosome; these are known as ribozymes. [24]
RNA plays a critical role in mediating every step of the cellular information transfer pathway from DNA-encoded genes to functional proteins. Its diversity of biological functions stems from
the ability of RNA to act as a carrier of genetic information and to adopt complex three-dimensional folds that create sites for chemical catalysis. Atomicresolution structures of several large
RNA molecules, determined by X-ray crystallography, have elucidated some of the means by which a global fold is achieved. Within these RNAs are tertiary structural motifs that enable the highly anionic double-stranded helices to tightly pack together to create a
globular architecture.[25]
>>> Below are exerpts from references [3] and [6]
Traditionally, RNA structure, like protein structure, is described at the sequence or primary structure level, the secondary, tertiary and quaternary levels. Initially, RNA motifs were identified and described at the sequence level as commonly occurring short sequences in functional RNAs, such as transfer RNA (tRNA) or ribosomal
RNA (rRNA). The variation in these sequences, within or between
RNAs, is often represented by an expanded alphabet describing a consensus sequence (e.g. GNRA where N is any nucleotide and R is a purine). An example of a sequence motif is the Shine-Dalgarno sequence of bacterial mRNAs or the Sm binding sites in some eukaryotic non-coding RNAs or the Codons start (AUG) and stop (UAA).
These conserved sequences are often given in the context of the predicted base-pairing structure (e.g. tetraloops) surrounding
them.
The next level of RNA structure is the base-pairing, or secondary structure, which identifies both the canonically base-paired regions (helices) and non-paired regions (loops). This structure
can sometimes be calculated quite accurately from sequence information,
usually through a combination of thermodynamic and comparative sequence analyses.
At the level of secondary structure, the RNA double helix is the fundamental motif.
Once helices are specified, other motifs become apparent, which at the level of secondary structure are classified as hairpin (or terminal) loops, internal loops (including bulges), and multi-helix or junction loops. However, this description of RNA structure is incomplete as it takes no account of non-Watson-Crick basepairing [14, 15] and most tertiary interactions that stabilize the 3D fold.
RNA tertiary structure describes the overall 3D conformation of a single molecule as determined by crystallography, NMR or modeling methods. The 3D structure is determined by long-range intramolecular interactions such as pseudoknots, ribose zippers, kissing hairpin loops, tetraloop/tetra loop receptor interactions, coaxial helices and other yet uncharacterized modes of interaction, and can be mediated by intermolecular interactions with ligands including metals and small molecules or other macromolecules (DNA, RNA or protein). This coordinate information is provided in both the Nucleic Acid Database and the Protein Data Bank (RCSB).
RNA structural motifs have been defined variously as ''directed and ordered stacked arrays of non-Watson-Crick base pairs forming distinctive foldings of the phosphodiester backbones of the
interacting RNA strands'' [8] and �\''a discrete sequence or combination of base juxtapositions found in naturally occurring RNAs in unexpectedly high abundance'' [5]. A comprehensive definition of an RNA structural motif should be based on and consist of not only base-pairing or secondary structure constraints, but a complete 3D description, including backbone conformation, all hydrogen-bonding and base-stacking interactions, and sequence preferences.
Examples of structural motifs (as defined according to LW nomenclature [14, 15]) are the Sarcin-ricin and E-loop motifs [7], C-loop and Kink turn motifs [9].
References
[1] E.Westhof and P. Auffinger. RNA Tertiary structure. Encyclopedia of Analytical Chemistry.
R.A.Meyers (Ed), pages 5222-5232, 2000.
[2] R.T. Batey, R.P. Rambo, and J.A. Doudna. Tertiary motifs in RNA structure and folding.
Angew. Chem. Int. Ed., 32:2326-2343, 1999.
[3] D.K. Hendrix, S.E. Brenner, and S.R. Holbrook. RNA structural motifs : building blocks
of a modular molecule . Quarterly Reviews of Biophysics, 38:221-243, 2005.
[4] S.R.Holbrook. RNA structure: the long and the short of it . Current Opinion in Structural
Biology, 15:302-308, 2005.
[5] P.B. MOORE. Structural motifs in RNA. Annual Reviews of Biochemistry 68, 287-300, 1999.
[6] N.B. Leontis, A. Lescoute, and E. Westhof. The building blocks and motifs of RNA
architecture. Current Opinion in Structural Biology, 16:1-9, 2006.
[7] N.B. Leontis, J. Stombaugh, and E. Westhof. Motif prediction in ribosomal RNAs -
lessons and prospects for automated motif prediction in homologous RNA molecules.
Biochimie, 84:961-973, 2002.
[8] N.B. Leontis and E. Westhof. Analysis of RNA motifs. Current Opinion in Structural Biology, 13:300-308, 2003.
[9] A. Lescoute, N.B Leontis, C. Massire, and E.Westhof. Recurrent structural RNAmotifs, Isostericity matrices and sequence alignments. Nucleic Acids Research, 33:2395-2409,
2005.
[10] S. Szep, J.Wang, and P.B. Moore. The crystal structure of a 26-nucleotide RNA containing a hook-turn. RNA, 9:44-51, 2003.
[11] A. Lescoute and E. Westhof. The A-minor motifs in the decoding recognition process. Biochimie, 88:993-999, 2006.
[12] P. Nissen, J.A. Ippolito, N. Ban, P.B. Moore, and T.A. Steitz. RNA tertiary interactions in the large ribosomal subunit: the A-minor motif. PNAS, 98:4899-4903, 2001.
[13] C.C. Corell, J. Beneken, M.J. Plantinga, M. Lubbers, and Y-L. Chan. The common and the distinctive features of the bulged-G motif based on a 1.04 -Aresolution RNA structure. Nucleic Acids Research, 31:6806-6818, 2003.
[14] N.B. Leontis, J. Stombaugh, and E. Westhof. Survey and summary. The non-Watson-Crick base pairs and their associated isostericity matrices. Nucleic Acids Research, 30:3497-3531, 2002.
[15] N.B. Leontis and E. Westhof. Geometric nomenclature and classification of RNA base pairs. RNA, 7:499-512, 2001.
[16] S. Lemieux and F. Major. RNA canonical and non-canonical base pairing types: a recognition
method and complete repertoire. Nucleic Acids Research, 30(19):4250-4263, 2002.
[17] P.J. Artymiuk, R.V. Spriggs, and P. Willett. Graph theoretic methods for the analysis of
structural relationships in biological macromolecules: Research articles. J. Am. Soc. Inf.
Sci. Technol., 56(5):518-528, 2005.
[18] C.M. Duarte, L.M. Wadley, and A.M. Pyle. RNA structure comparison, motif search
and discovery using a reduced representation of RNA conformational space. Nucleic
Acids Research, 31(16):4755-4761, 2003.
[19] A. Harrison, D.R. South, P. Willett, and P.J. Artymiuk. Representation, searching and
discovery of patterns of bases in complex RNA structures. Journal of Computer-Aided
Molecular Design, 17(8):537-549, 2003.
[20] E. Hershkovitz, E. Tannenbaum, S.B. Howerton, A. Sheth, A. Tannenbaum, and L.D.
Williams. Automated identification of RNA conformational motifs: theory and application
to the HM LSU 23S rRNA. Nucleic Acids Research, 31:6249-6257, 2003.
[21] D. Oranit, R. Nussinov, and H. Wolfson. ARTS: alignment of RNA tertiary structures. Bioinformatics, 21(suppl 2):ii47-53, 2005.
[22] M. Sarver, C.L. Zirbel, Jesse Stombaugh, Ali Mokdad, and Neocles B. Leontis. FR3D: Finding local and composite recurrent structural motifs in RNA 3D structures. Journal
of Mathematical Biology, 56(1-2):215-252, 2008.
[23] L.M. Wadley and A.M. Pyle. The identification of novel RNA structural motifs using COMPADRES: an automated approach to structural discovery. Nucleic Acids Research,
32:6650-6659, 2004.
[24] Wikipedia, the free encyclopedia
[25] R.T. Batey et al. Tertiary Motifs in RNA Structure and Folding. Angew. Chem. Int. Ed. 1999, 38, 2326- 2343.
![]() |
![]() |
![]() |